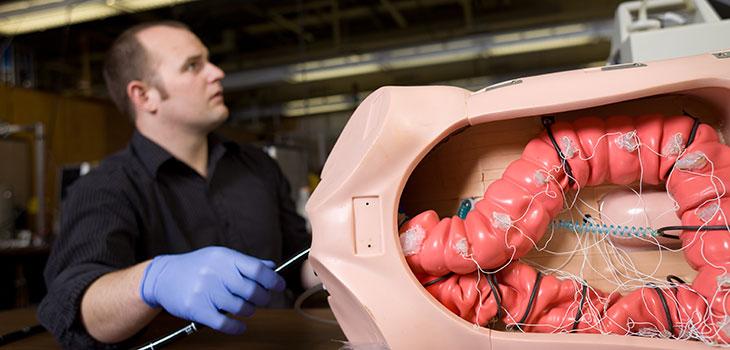
Biomechanics
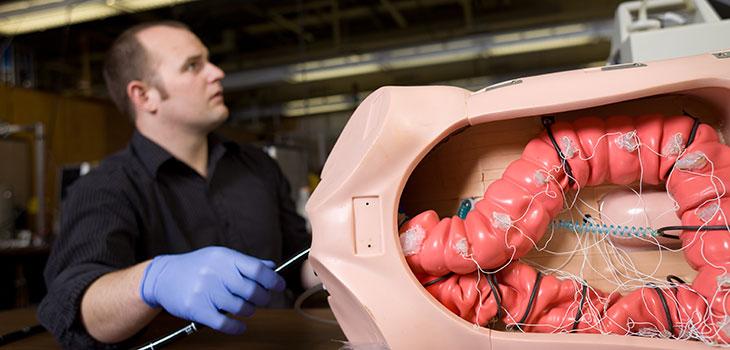
Biomechanics marries biological sciences and mechanical engineering to understand human physiology and develop therapeutic mechanical devices and diagnostic tools. Faculty in this research area apply the laws of mechanics to virtual human musculoskeletal anatomy and physiology to study and design prosthetic devices such as artificial joints.
Robotics, Haptics, and Biomechanics Lab
Robert Williams II, professor of mechanical engineering, studies the human body and its motion and sensors to improve the design, analysis, and control of robotic manipulators. The Baxter Robot system is an anthropomorphic robot with two 7-dof arms, compliant to work with humans; it is a collaborative robot (cobot). Haptics involves force and touch feedback to improve the realism of virtual simulations for training, education, and gaming. Dr. Williams is also interested in the rigid-body motion of the human body.
Impact Mitigation in Prosthetic Devices
Muhammad Ali, associate professor of mechanical engineering, seeks to exploit effective energy absorption characteristics of functionally graded biomaterials. He researches applications of graded honeycomb structures for impact mitigation in prostheses, particularly artificial hip and knee joints. The idea of utilizing functionally graded materials with slanted cell shapes was acquired by observing that banana peel cross-sections, which contain honeycomb shaped graded structure, protect the internal soft core of bananas from external impacts. In collaboration with other researchers, Ali has explored low-, medium-, and high-impact responses of homogeneous, composite, and crushable foam filled honeycomb functionally graded structures and developed mathematical models that enable the design of graded honeycomb structures by defining the number of cells, size, and shape of cells, type of cell patterns for a given thickness, loading conditions, and material types.
Improved Computer Simulations of Bone Fracture
The ability to better predict the fracture of bone may improve prosthetic design and clinical predictions of fragility in the elderly. Moving beyond the limitations of DEXA scans, John Cotton, associate professor of mechanical engineering, creates models from CT scans and performs finite element analysis (FEA) to capture local geometry and material effects. Using both commercial FE solvers and code written in house, simulations include local yielding and fatigue effects. Additional information on bone material is gained through use of CBMT technology developed at Ohio University, which estimates long bone stiffness through mechanical vibration in a non-invasive test. Future work emphasizes demonstrating best practices for accuracy across anatomic locations and automating processes to move mechanical bone simulation from bench top to clinic.